What is Raman spectroscopy?
Raman spectroscopy is a non-destructive materials analysis technique in which a monochromatic light source, usually a laser, is directed onto a sample of the materials being analyzed. The interplay of the light and the vibrations of the molecules in the materials generate spectra that can be used to identify materials, characterize molecular structure, assess morphology, and observe dynamic processes. Raman spectroscopy requires little sample preparation and can be used in situ or ex situ.
Obtaining Raman spectroscopy measurements was a time consuming, complex process. As a result of advances in Raman spectroscopy, the technique now delivers much higher sensitivity, better resolution, and a broader range of battery R&D applications. What’s more, current Raman spectroscopy instruments are relatively quick and easy to use, allowing even those with limited science expertise to operate them effectively.
How is Raman spectroscopy used in battery R&D?
Raman spectroscopy plays an important role in advancing battery technology by providing critical information that can be used to analyze battery components, such as cathode, anode and electrolyte materials. Cathode and anode materials degrade over time, but Raman spectroscopy provides insights into their molecular structures, helping researchers measure degradation rates. Reducing the degradation rates of these materials is a key step in developing better batteries.
Raman spectroscopy helps advance understanding of the properties of both liquid and solid electrolytes, including ion transport mechanisms, phase changes, and chemical interactions. This information is indispensable for creating more efficient and stable electrolytes.
A versatile tool, Raman spectroscopy can help evaluate the degree of interaction among electrolyte ions within solutions and polymeric substances. These interactions directly impact battery performance. Additionally, the technique offers valuable insights into the composition of polymer matrices and the ways in which additives can influence their crystalline structure, another factor impacting battery performance.
An extension of Raman spectroscopy, Raman mapping and imaging helps analyze the distribution of materials on electrode surfaces, or across cross-sections. The data obtained can be quantified, giving metrics such as fraction estimates and particle statistics.
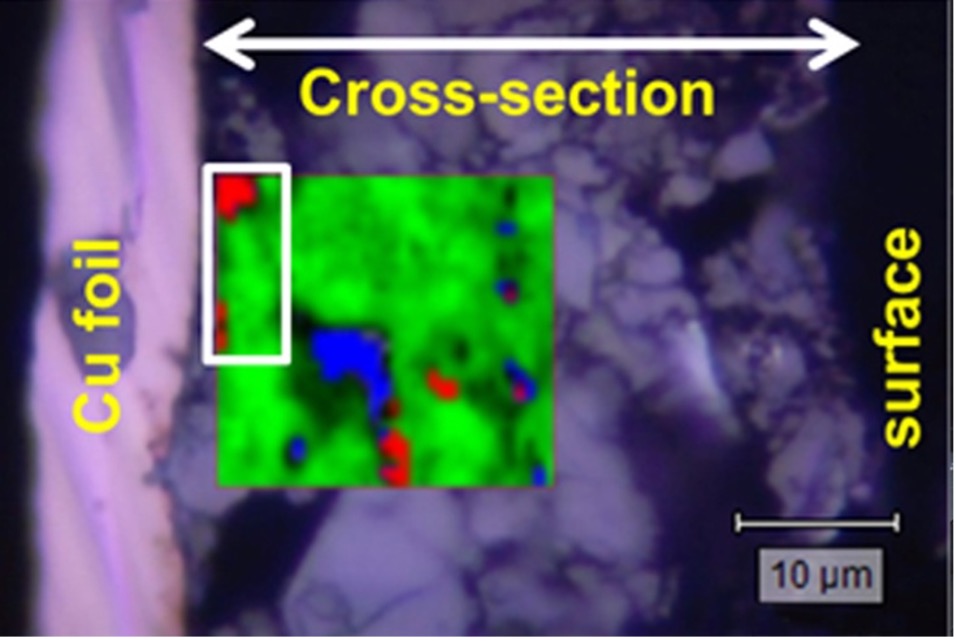
Detecting low concentrations of binder. Raman map of an anode (superimposed on an optical microscope image). The colours represent: SBR styrene-butadiene rubber binder (red); graphite (green); acetyl black (blue). The relative concentrations, as determined by the map, are, respectively: 1%, 97%, and 2%.
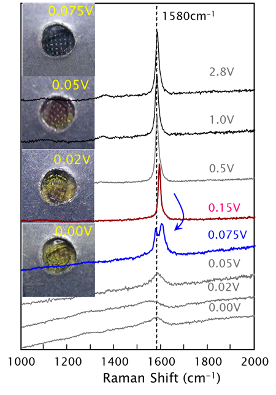
Operando studies of an anode. As the potential is changed, the anode’s appearance changes. The graphite G-band Raman peak also changes, indicating intercalation of lithium (shifting the peak to higher wavenumbers) and then a peak-splitting reflecting the intercalation penetrating to interior layers, rather than just the boundary layers. Data courtesy of Prof. Y. A. Kim, Shinshu University, Japan.
In situ analysis of batteries is conducted with batteries that are fully assembled and in operation. With Raman spectroscopy instruments, in situ analysis can provide information on chemical reactions that occur as batteries are charged and discharged, helping in the development of new battery materials.
After new materials are produced and prototype batteries are produced with them, it is essential to determine how these materials impact performance, and what it is that makes them either better or worse than their predecessors. This is when ex situ analysis is done, a process requiring disassembly of the batteries and analysis of their components in an inert environment using Raman spectroscopy instruments.
Understanding the Solid-Electrolyte Interphase (SEI) layer is essential for battery safety and performance. Raman spectroscopy is used to analyze the SEI layer’s composition and thickness, helping to minimize issues like capacity fading and dendrite formation. Raman spectroscopy can also be used to study the thermal behaviour of battery materials and investigate safety concerns, such as the risk of thermal runaway.
Raman spectroscopy can also be employed for quality control in battery manufacturing to ensure that materials and components meet the desired specifications, preventing defects and inconsistencies.
Supporting electric vehicle battery range performance research
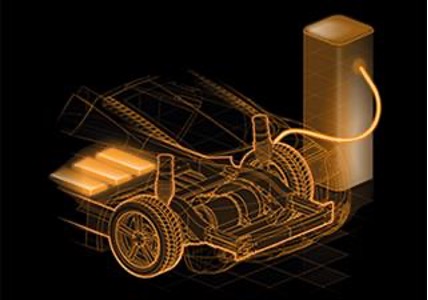
Automotive R&D is increasingly focused on new propulsion technologies for the next generation of hybrid and electric vehicles (EV). At the heart of EV product development is the pursuit of extended range through motor efficiency and battery effectiveness.
Renishaw Raman technologies offer a non-destructive method of monitoring and imaging battery chemistry so that the most suitable materials can be developed and their performance limits understood. Renishaw’s inVia™ confocal Raman microscope, for example, enables automotive battery manufacturers to examine battery chemistry under a range of operating conditions (such as fast-charging and extremes of temperature) to see how the battery reacts and work out how to improve its efficiency.
Apart from R&D of lithium-ion batteries widely used to power electric vehicles, Raman spectroscopy contributes to the development of emerging, next-generation battery technologies, such as lithium-sulfur and solid-state batteries, by helping researchers investigate the unique challenges and materials associated with these systems.
In summary, Raman spectroscopy is an invaluable analytical tool for battery R&D, providing critical information about the characterization, optimization, and safety assessment of various battery components, all of which are essential for advancing battery applications.
Renishaw Raman spectroscopy instruments
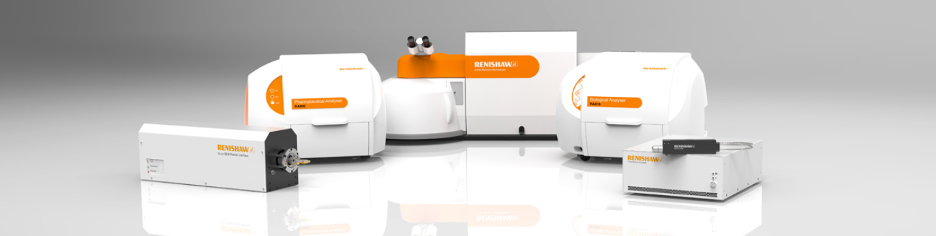
Renishaw produces a wide range of Raman spectroscopy instruments, including research-grade microscopes, routine bench-top analysers, transportable fibre-optic analysers and combined (hybrid) systems. These state-of-the-art instruments help researchers gain insights across a range of battery applications. Click here to learn more.
Contact
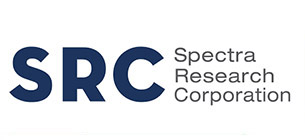
Click here to contact SRC and speak directly with our experts on Renishaw Raman Spectroscopy Instruments.